December 8, 2020 Webinar Speakers
- jawschem
- Nov 30, 2020
- 6 min read
Our next webinar will occur on Tuesday December 8th, 2020 at 11am EST/4pm GST. Please note that every week the seminar time will alternate to accommodate presenters and attendees from different time zones. Sign up on our mailing list to receive the zoom link!
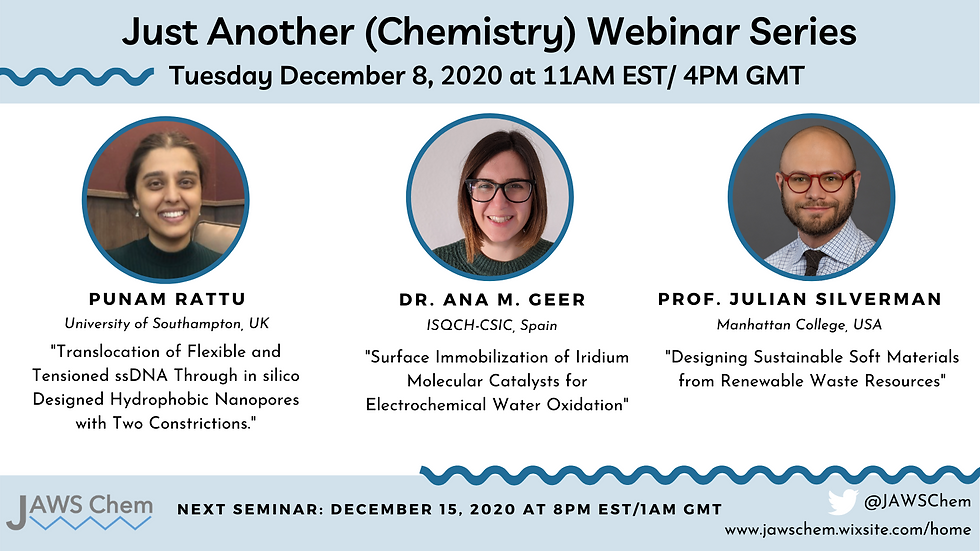
Our featured speakers this week are Punam Rattu (PhD graduate student; University of Southampton, UK), Dr. Ana M. Geer (Postdoctoral researcher; ISQCH-CSIC, Spain), and Prof. Julian Silverman (Assistant professor; Manhattan College, USA).
LEARN MORE ABOUT THE SPEAKERS AND THEIR TALKS BELOW

PUNAM RATTU
Biography: Punam Rattu is a PhD student in Syma Khalid's research group based in the Computational Systems Chemistry sub-section of theSchool of Chemistry, University of Southampton (UK). Punam completed her master's degree in Biomedical Sciences before pursuing computational research aiming to optimise nanopore devices used for DNA sequencing. Her work is funded by Oxford Nanopore Technologies.
Title of Talk: Translocation of flexible and tensioned ssDNA through in silico designed hydrophobic nanopores with two constrictions.
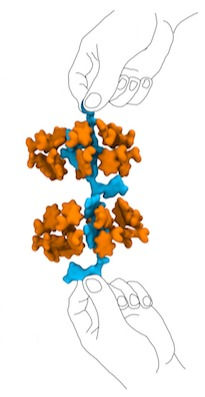
Abstract: DNA sequencing using nanopores is now a well-established ‘next generation sequencing’ method. Over the years, research has focused towards optimising the apertures within the membrane with the aim of controlling conformation and translocation rate of DNA to improve the resolution of the devices. Experiments involving protein engineering of S. aureus toxin α-hemolysin (a heptamer that forms a 14-stranded β-barrel in the membrane) revealed that basic residues introduced in the pore lumen slowed down the translocation of ssDNA. Subsequent simulation studies of simplified models of the α-hemolysin pore region showed that the strong electrostatic interactions between the basic residues and the acidic phosphate groups of the DNA led to transient tethering of the DNA to the pore, which resulted in undesirable conformations that may impact on the accuracy of the read.[1] Model pores with central hydrophobic constriction region maintained DNA in a largely linear conformation, resulting in bases translocating through the constriction in the correct order.[2] Taking this forward, and inspired by experimental work showing the potential advantages of nanopores with two constrictions for DNA sequencing, we have designed model pores with two hydrophobic constrictions (14- and 16-stranded β-barrels) to explore the impact of pore geometry and chemical nature of the constrictions on DNA translocation rate and conformations using MD simulations. Our results show that DNA entry into these model pores is strongly influenced by the pore width under an applied electric field. However, no correlation was found between pore width and translocation rate when DNA is inside the pore. DNA translocation was slowed down by aromatic residues in the constrictions, which interacted with DNA and also caused the strand to deviate from its linear conformation. Overall, our pores with phenylalanine constrictions are the most promising as they slowed down DNA translocation whilst not causing too many conformational changes. References: [1] P.J. Bond, A. T. Guy, A. J. Heron, H. Bayley and S. Khalid, Biochemistry, (2011), 50, 3777-3783. [2] T. Haynes, I. P. S. Smith, E. J. Wallace, J. L. Trick, M. S. P. Sansom and S. Khalid, ACS nano, (2018), 12, 8208-8213.

DR. ANA M. GEER (on twitter @atinageer)
Biography: Dr. Ana M. Geer recently became a Juan de la Cierva Fellow at the Institute for Chemical Synthesis and Homogeneous Catalysis (ISQCH-CSIC). Her research interests lie in the development of molecular catalysts and materials for chemical transformations of relevance to energy processes. She obtained her PhD at the University of Zaragoza on the synthesis of Rh and Ir complexes in stoichiometric and catalytic transformations. Afterwards, she worked as a research fellow in the University of Nottingham with Prof. Kays studying catalytic applications of 1st row transition metal complexes and with Prof. Brent Gunnoe in electrochemical water oxidation
Title of Talk: Surface immobilization of iridium molecular catalysts for electrochemical water oxidation
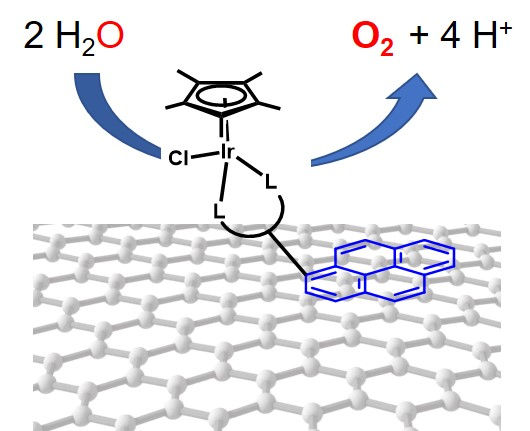
Abstract: It is increasingly important to find a clean, renewable energy source to replace fossil fuels.[1] Artificial photosynthesis, which converts solar energy to chemical fuels (H2) or CO2 reduction products (e. g., CH3OH, CO, HCOOH), has been proposed a promising alternative in which renewable electrical power can be turned into storable fuel.[2-4] Water splitting to produce hydrogen fuel (2H2O → O2 + 4e- + 4H+; 2H+ + 2e- → H2) is a widely investigated reaction in artificial photosynthesis. The oxygen evolution reaction (OER) necessary for water splitting has several drawbacks, including a large overpotential and sluggish kinetics due to the complex multi-electron and multi-proton transfer. Therefore, efficient and stable water oxidation catalysts which can convert water to dioxygen at relatively low overpotentials are key for any water splitting device.
Electrochemical water oxidation with molecular catalysts has been largely studied due to the advantage of catalysts with tuneable structures which allow of studying for understanding of structure/activity relationship, difficult to achieve with heterogenous catalysts.[5] Yet, homogeneous electrocatalysts often suffer from limitations including catalytic activity limited by diffusion to the electrode and a lack of stability of the catalyst.[6] Immobilization of molecular catalysts on supports for electrocatalysis is a cutting-edge strategy that can potentially address these drawbacks while facilitating selective substrate oxidation using well-defined catalytic active sites.7, 8
In this context, we have prepared Cp*Ir molecular catalysts attached to ordered mesoporous carbon (OMC) using non-covalent methodologies and tested the materials for electrocatalytic water oxidation leading to an increase in the stability of the complexes in acidic media. Highlights of these strategies along with catalytic results will be discussed.
[1] Agency, I. E., World Energy Outlook 2018. 2018. [2] Zhang, B.; Sun, L., Artificial photosynthesis: opportunities and challenges of molecular catalysts. Chem. Soc. Rev. 2019, 48 (7), 2216-2264. [3] Concepcion, J. J.; House, R. L.; Papanikolas, J. M.; Meyer, T. J., Chemical approaches to artificial photosynthesis. Proc. Natl. Acad. Sci. U.S.A 2012, 109 (39), 15560-15564. [4] Lewis, N. S.; Nocera, D. G., Powering the planet: Chemical challenges in solar energy utilization. Proc. Natl. Acad. Sci. U.S.A 2006, 103 (43), 15729-15735. [5] Blakemore, J. D.; Crabtree, R. H.; Brudvig, G. W., Molecular Catalysts for Water Oxidation. Chem. Rev. 2015, 115 (23), 12974-13005. [6] Bullock, R. M.; Das, A. K.; Appel, A. M., Surface Immobilization of Molecular Electrocatalysts for Energy Conversion. Eur. J. Chem. 2017, 23 (32), 7626-7641. [7] Garrido-Barros, P.; Matheu, R.; Gimbert-Suriñach, C.; Llobet, A., Electronic, mechanistic, and structural factors that influence the performance of molecular water oxidation catalysts anchored on electrode surfaces. Curr. Opin. Electrochem. 2019, 15, 140-147. [8] Hickey, D. P.; Minteer, S. D., From Biological to Biomimetic: Immobilizing Electrocatalysts for H2/O2 Fuel Cells. Joule 2019, 3 (8), 1819-1821.

PROF. JULIAN SILVERMAN (on twitter @heterocat)
Biography: Julian R. Silverman, PhD, is an Assistant Professor in the Chemistry and Biochemistry department at Manhattan College in New York City. His research focuses on developing and characterizing sustainable soft materials from waste biomass with a focus on gels and plastics for personal care products. He is the current Director of the Center for Urban Resilience and Environmental Sustainability (C.U.R.E.S.) where he works to develop green chemistry and toxicology curricular materials for scientists and students from all disciplines. In his spare time he's found on twitter (@heterocat), playing rouge-like video games, and discussing lignin processing with his two rabbits.
Title of Talk: Designing Sustainable Soft Materials from Renewable Waste Resources
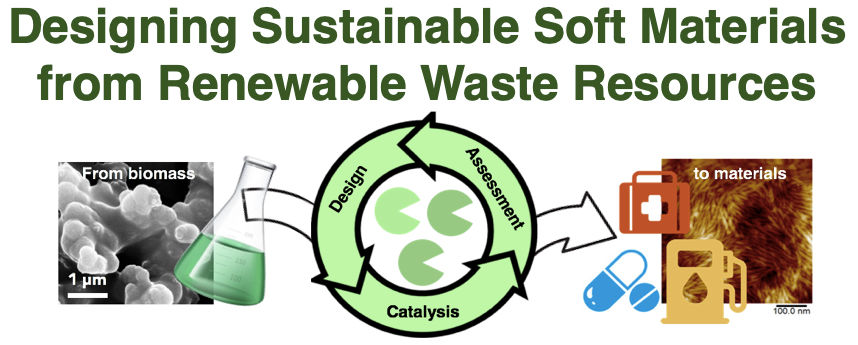
Abstract: The next generation of materials will have advanced functionalities such that they can be more efficient and effective in their variety of applications. To meet these needs and address important environmental concerns soft materials may be derived not only from renewable chemicals but come from abundant waste resources. Examining waste resources we can highlight and leverage chemical components such as aromatics from lignins, hydrocarbon chains from frying oils, and carbohydrates from sugars and polymers. By joining these molecules together we can create hybrid molecules with the functionality of both components that are stimuli responsive and predictably respond to heat, light, pH, and mechanical forces. One example includes amphiphiles synthesized from sugars and lipids to create fluorescent gellants to detect radiation. One challenge of working with waste resources is processing and isolating valuable chemicals, and identifying the appropriate applications for these products. This talk will focus on several examples where local waste resources are sourced and then processed into advanced materials including polymers from lignin and gels from spent cooking oil. Traditional spectroscopic and chromatographic methods are used to characterize these materials, which are then targeted for specific applications (e.g. personal care products, plastics and coatings). Life-cycle techniques will be explored to quantitatively address whether these products are more sustainable. Using these ideas we can realize that in nature there is no such thing as waste, and that one person’s chemical trash can be creatively transformed into material treasures.
Comments